BSB Seminar Series: The Antimalarial Peroxide Artemisinin and Derivatives: Mechanism of Action - The "Heme Activation" Hypothesis vs. Oxidant Properties
Over 50 years ago, the active principle 青蒿素 qīng hāo sū or artemisinin was isolated in pure crystal-line form from the leaves of the Chinese traditional herb 青蒿 qīng hāo - Artemisia annua - by Chinese groups working under the remarkable Project 523 during the latter stages of the Chinese Cultural Revolution.
Speakers
Event series
Content navigation
Description
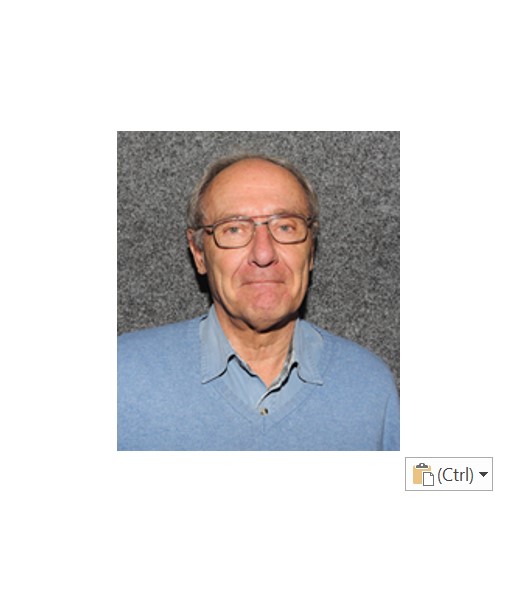
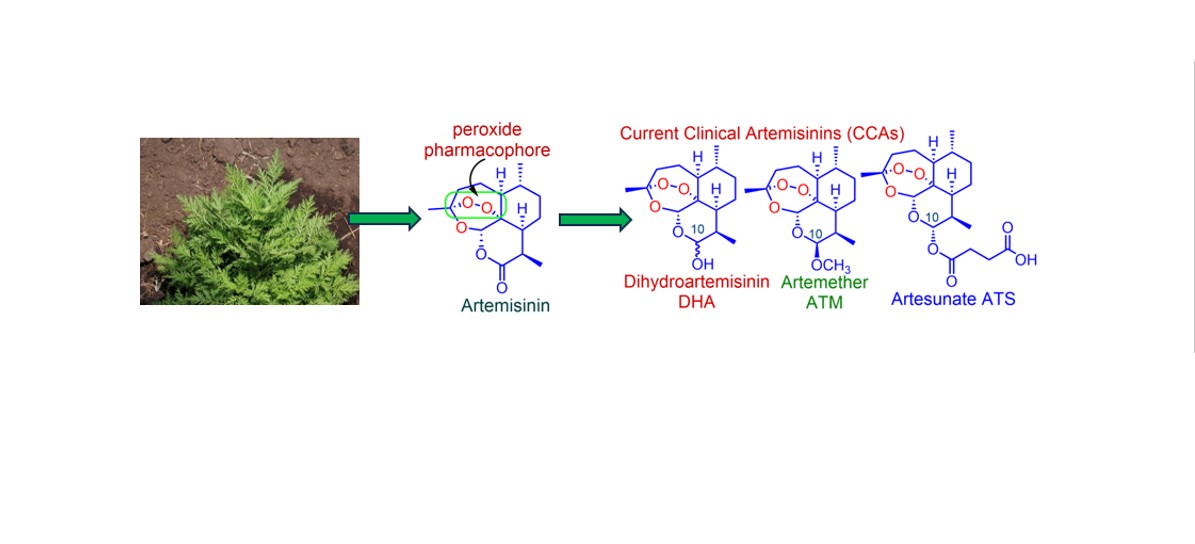

The Antimalarial Peroxide Artemisinin and Derivatives: Mechanism of
Action - The "Heme Activation" Hypothesis vs. Oxidant Properties
by Richard K. Haynes & Ho Ning Wong
ABSTRACT
Over 50 years ago, the active principle 青蒿素 qīng hāo sū or artemisinin was isolated in pure crystal-line form from the leaves of the Chinese traditional herb 青蒿 qīng hāo - Artemisia annua - by Chinese groups working under the remarkable Project 523 during the latter stages of the Chinese Cultural Revolution. Artemisinin is a unique sesquiterpene containing the active peroxide pharmacophore embedded in a 1,2,4-trioxane ring that is responsible for its biological activities [1]. Because of poor physicochemical properties, artemisinin was converted by Chinese groups into dihydroartemisinin (DHA), artemether (ATM) and artesunate (ATS). However, these derivatives do have notably short pharmacological half-lives [ATM t1/2 ~1.3 h → DHA; ATS t1/2 ~14 min → DHA; DHA t1/2 ~1 h → inert decomposition products + phase II metabolism], but despite this, after 50 years, these are still the only clinically-used artemisinin derivatives (CCAs). The CCAs are now used in artemisinin combination therapies (ACTs) against malaria with quinoline-based antimalarial drugs such as mefloquine, piperaquine, lumefantrine and others. Use of ACTs dominate current malaria chemotherapy: 4 billion treatment courses were prepared between 2010 and 2022 and 65% of the 249 million non-severe malaria cases in 2022 were treated with ACTs [2].
Development of new derivatives is urgently required, given especially the problem of emergence of malaria parasites that are increasingly tolerant of ('resistant' to) the CCAs. Such an activity must take into account pharmacokinetic aspects [3] and knowledge of mechanism of action against the malaria parasite [4]. Artemisinins are held to undergo 'bioactivation' by heme-Fe(II) [5], or by labile Fe(II). The 'bioactivation' entails reductive scission of the peroxide to generate C-radicals which 'alkylate' intraparasitic targets (Scheme). But does this auspiciously popular hypothesis [6,7] have any connection with reality? The C-radicals are feeble 'alkylating' agents [8], certain analogues cannot generate such C-radical (Scheme), and the intraerythrocytic malaria parasite is in an aerobic environment [9] wherein C-radicals will react at a diffusion-controlled rate with O2. In further contradiction of the hypothesis, increasing the O2 content over parasite cultures enhances activity of the artemisinin [10,11]. In control experiments, heme-Fe(II) partially degrades the current artemisinin derivatives, although highly anti-malaria-active amino-artemisinins are largely inert [12]. Endogenous heme-Fe(II) is converted into the unreactive heme-Fe(II)-CO complex when malaria parasites are cultured in an atmosphere enriched in CO. Those artemisinins degraded by heme-Fe(II) now display enhanced activities, whereas the amino-artemisinins display unaltered activities [11,13]. A detailed review of these experiments and others in relation to the labile-Fe(II) and heme-Fe(II) activation hypotheses is given elsewhere [14].
It should be recognized that artemisinins as peroxides do possess overt oxidizing capabilities. Indeed, in model experiments, they are shown to rapidly oxidize reduced flavin cofactors of critical flavin disulfide reductases and other flavoenzymes required for maintaining intra-parasitic redox homeostasis (Scheme) [15]. This is compatible with the ability of artemisinins to perturb redox homeostasis through enhancing generation of reactive oxygen species (ROS) [11,16], not just within the malaria parasite, but in distinct intracellular systems associated with other targets against which artemisinins are active. The hypotheses and details of the associated experiments are critically discussed in relation to established biological activities of artemisinins against the malaria parasite and these other targets.
References
1. Cui L, Su X.Z. Expert Rev. Anti Infect. Ther. 2009, 7, 999; doi: 10.1586/eri.09.68
2. World Malaria Report 2023, Geneva: World Health Organization, 2023; Dhorda M, Kaneko A, Kapologwe H, Assefa A, White NJ, von Seidlein L et al. Science 2024, 385, 252-254; doi 10.1126/science.adp5137.
3. Watson DJ, Wong HN, Haynes RK et al. Front. Pharmacol. 2024, 14, 1308400; 10.3389/fphar.2023.1308400.
4. Kavishe RA, Koenderink JB, Alifrangis M. FEBS J. 2017, 284, 2579-2591; doi: 10.1111 /febs.14097.
5. Leiserowitz L et al. Scientific Rep. 2017, 7, 7610; doi: 10.1038/s41598-017-06650-w; Roepe PD, de Dios AC. Inorg. Chim. Acta 2019, 496, 119029; doi: /10.1016/j.ica.2019 .119029.
6. For a recent review, see Shukla M, Rathi K, Hassam M et al. Med Res Rev. 2024, 44, 66‐137; doi:10.1002/med.21979.
7. Wittlin S., Mäser P. ACS Infect. Dis. 2021, 7, 2777; doi: 10.1021/acsinfecdis.1c00118.
8. Hawkins CL, Davies MJ. Biochim. Biophys. Acta 2001, 1504, 196-219; doi: 10.1016/ S0005-2728(00)00252-8; Nauser T, Gebicki JM. Biochem. Biophys. 2017, 633, 118-123; doi: 10.3390/ijms23010396.
9. Tiwari S, Mishra N et al. Parasitology Res. 2021, 120, 423-434; doi: 10.1007/s00436-021-07051-9.
10. Krungkrai SR, Yuthavong Y. Trans. R. Soc. Trop. Med. Hyg. 1987, 81, 710-714;
doi: 10.1016/0035-9203(87)90003-4.
11. Parapini S, Monti D et al. FEBS Letters 2004, 575, 91-94; doi: 10.1016/j.febslet.
2004.08.039
12. Haynes RK, Ho WY, Robinson BL et al. Angew. Chem. Int. Ed. 2004, 43, 1381-1385;
doi: 10.1002/ange.200352343.
13. Coghi P, Haynes RK, Monti D et al. ChemMedChem 2009, 4, 2045-2053;
doi: 10.1002/cmdc.200900342.
14. Haynes RK,Coghi P, Monti D et al. Infect Disord Drug Targets, 2013, 13, 217-277;
doi: 10.2174/1871526513666131129155708.
15. Haynes RK, Wong HN, Monti D et al. ChemMedChem 2012, 7, 2204-2226;
doi: 10.1002/cmdc.201200383 and previous references.
16. Gopalakrishnan AM, Kumar N. Antimicrob. Agents Chemother. 2015, 59, 317–325; doi: 10.1128/aac.03663-1
BIOGRAPHY
Prof. Haynes obtained his Ph.D. in organic chemistry from the University of Western Australia and conducted postdoctoral work at the University of Karlsruhe Germany as Gillette International Fellow, and then at Imperial College, London. Previous academic appointments were at Monash University, University of Sydney, the Hong Kong University of Science and Technology (HKUST) (1993-2011), and the Centre of Excellence for Pharmaceutical Sciences, North-West University (NWU), South Africa (2011-2023). He is currently appointed as Senior Principal Research Fellow, Rural Health Research Institute, Charles Sturt University (March 2023-).
Whilst at the University of Sydney, Prof. Haynes participated in the Australian Academy of Science-Chinese Academy of Science Exchange Programs enabling visit to Chinese universities and research institutes in 1988 and 1990. Consultation on development of the artemisinin derivative artemether with Rhône Poulenc Rorer, Paris, enabled travel to China to visit artemisinin extraction and manufacturing facilities in Kunming, Guilin and Guangzhou (1991-94). Whilst at HKUST, Prof. Haynes also was consultant with the AusAID-ACIL Vietnam Malaria Control Programme that enabled visits to Vietnam and malaria drug production facilities (1993-96). He was an external member of the CHEMAL and then the Drug Discovery Research Committees of Tropical Diseases Research (TDR) of the World Health Organization (WHO), Geneva (1993-2002), collaborated on the TDR/WHO artesunate antimalarial drug development project (1998-2008), and participated in the ICS-UNIDO malaria workshop in Trieste (2009). Whilst at NWU, he was an A-rated researcher with the South African National Research Foundation (2014-2020). Prof. Haynes is a Fellow of the Royal Society of Chemistry, Fellow of the Royal Australian Chemical Institute, and member of the American Association for the Advancement of Science and the American Chemical Society. Prof. Haynes has 214 publications in the primary scientific literature; other outputs include patents, book chapters, and >60 consulting reports for TDR/WHO, government agencies and industry. He is the 6th most co-cited author in artemisinin area, and 3rd in link strength.
Research activities involve drug development as directed from an organic chemistry/medicinal chemistry aspect. The first area focuses on new derivatives of the Chinese peroxidic antimalarial drug artemisinin, the active principle of the traditional Chinese herb qīng hāo 青篙 (Artemisia annua). One derivative was the non-neurotoxic artemisone developed under a research and contract agreement between HKUST and Bayer AG, Germany (1995-2007). Artemisone successfully completed a clinical Phase IIa trial in non-severe malaria patients. Artemisone and related compounds are highly active against other apicomplexan parasites including important veterinary parasites that cause babesiosis, neosporosis and toxoplasmosis, against the non-apicomplexan parasites that cause schistosomiasis and leishmaniasis, and against the ulcerogenic bacterium Helicobacter pylori. It is also potently active against cytomegalovirus, an important infection in immunocompromised individuals, a usage for which artemisone has been patented. In November 2017, artemisone was listed with the FDA as an orphan drug for treatment of malaria and viral diseases. Ongoing activities focus on mechanism of action of artemisinin, and development of rational combinations of new artemisinins with other structurally distinct drugs based on understanding of the mechanism of action of each of the drug components for treatment of malaria, other parasitic diseases including the diseases of veterinary importance listed above, cancer and bacterial diseases including tuberculosis. For the last, emphasis is on developing drugs that maintain adequate sterilizing capacity within the diverse microenvironments inhabited by the causative pathogen Mycobacterium tuberculosis.
Location
Please note: this seminar will be held in the Eucalyptus Seminar Rm and via Zoom, details are included below.
Eucalyptus Seminar Room, S205,
Level 2, RN Robertson Bldg (46)
Please click the link below to join the webinar:
https://anu.zoom.us/j/88695849855?pwd=iA0boLxaiIbPiOk8aK5bm5F2Q9coPK.1
Webinar ID: 886 9584 9855
Passcode: 810334
Canberra time: please check your local time & date if you are watching from elsewhere.